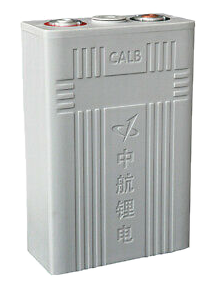
- Key properties of lithium batteries
- Batteries versus Cells
- How lithium cells work
- Example specifications
- Approaching and exceeding the limits
- Battery Management Systems (BMS)
- Discharge termination
- Charge termination
- Dual bus topology
- Cell balancing
- Terminology
If you are like most cruising sailors you probably hate your lead-acid batteries. They age quickly, the number of cycles you can get out of them is very limited, they can not be deep discharged without aging them even faster, they are heavy, and recharging them to 100% takes hours. And now you have heard so many nice things about lithium batteries that you can’t wait to get rid of these pesky lead-acid batteries and replace them by lithium batteries.
Well, not so fast. It is true that lithium batteries solve most of the shortcomings of lead-acid batteries, but lithium batteries introduce a new set of problems in return.
Key properties of lithium batteries
In this article we will discuss "Lithium batteries". In fact there is a whole family of "Lithium batteries", all with a specific chemistry tailored for a specific use, optimized for weight, size, voltage and/or temperature range, etc. For use on sailing boats, only LiFePO4 (also sometimes referred to as LFP) is a sensible choice. In this article read "LiFePO4" wherever we write "Lithium". The statements made might not apply to other types of Lithium batteries.
Let’s look for a moment to a few key properties of lithium batteries, which make them so different from lead-acid batteries:
Lithium batteries don't stop charging by themselves
Lithium batteries absorb current very well. While lead-acid batteries become very reluctant to accept a charge when they are about 80% charged, lithium batteries keep accepting the full available current up to the end, so they charge very fast and make optimal use of the available charge current. In fact, they keep accepting the full current even after the end, when they are already completely fully charged. To protect the lithium cells against destroying themselves the charge current must be somehow terminated once the cells are fully charged. We will come back to that in a moment.
Satellites
The lifetime of satellites is often determined by the lifetime of their lithium battery banks and for this reason the lithium batteries are only cycled between 40% and 60% SOC, which is rewarded by a lifetime often exceeding 40,000 cycles! (No, this is not a typo, a complete day-night cycle of a typical low orbit satellite only takes about 2 hours, so with 40,000 cycles this amounts to about 9 years of service). Of course, the power consumption and solar energy production of satellites is very predictable and they don’t have to cope with shade on the solar panels or unexpected power consumption, so remaining in this state-of-charge window is relatively easy to achieve. This example just illustrates that the amount of cycles can be practically infinite as long as the average state of charge is kept very low.
Lithium batteries degrade when fully charged
Lithium batteries love to cycle, but they hate to remain in a fully charged state. Lithium batteries are best kept in a discharged state of around 40% energy left. At a higher State Of Charge (SOC) the chemical components inside the battery become more reactive, especially at a SOC higher than 80% and this becomes even worse at tropical temperatures. The higher the average charge state of the lithium battery, the shorter its lifetime.
It is not the amount of cycles that determine the aging of lithium batteries but the amount of time kept at a highly charged state.
Many people learn that the hard way when they keep their laptop plugged in all the time and discover that the "almost never used" Lithium battery after only one year hardly keeps a charge anymore. Indeed, this is the complete opposite of lead-acid batteries which will live almost forever when kept in a fully charged state all the time but will die rather quickly when kept heavily discharged for a prolonged time.
The voltage curve is nearly flat
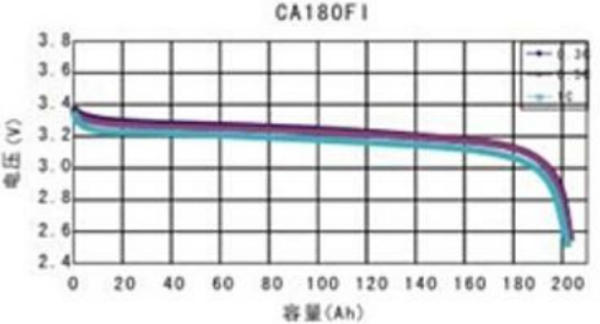
The charge/discharge voltage curve of a lithium cell is very different than the curve of a lead-acid cell. Very notable is the almost flat line between 10% and 90% SOC and the speed at which voltages rise or drop at the ends of the curve, and the relatively small difference between the normal voltage and damaging voltages.
Since a battery consists of multiple cells and individual cells never have exactly the same capacity, it is most likely that cells never reach the end of the curve at the same time. The problem here is that if only one cell is in the danger zone the overall voltage of the battery is still within the normal range! This makes it impossible to use voltage regulation to terminate the charge or discharge. We will explain that in more detail in chapter Voltage Based Charge Regulation. The only correct way is to look at cell voltages rather than battery voltage and terminate the charge and discharge by disconnecting battery from the respective charge sources or loads. This is explained in the chapters Charge termination and Discharge termination.
Batteries versus cells
Batteries are assembled from cells. The voltage of a cell is determined by the chemical process which delivers electrical energy, which is about 2 Volts for a lead-acid cell and 3.2 Volts for a lithium cell. So for a 12 Volt system you need 6 lead-acid cells, or 4 lithium cells in series.
An important thing to realize is that when cells are connected in series they form a circuit where the current through all these cells is always exactly the same, even when one cell is full and another one is empty.
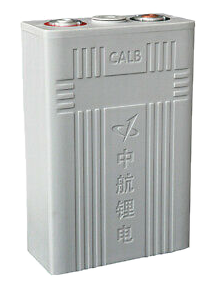
Lead-acid batteries are usually not assembled from individual cells but the cells are integrated in the undividable solid box we call a "battery". Because of the way in which lead-acid batteries work, it is enough to observe the voltage of the whole battery; most often the battery has only two poles so it is impossible to measure the voltages of the individual cells. Lithium batteries however are assembled from individual cells and often you just buy the individual cells and connect them together to form a battery-bank. The manufacturers don't talk about battery voltages but just give the specifications for the cells they manufacture. Because of the voltage curve of lithium cells the battery voltage is meaningless, so it is common when dealing with lithium batteries to talk instead about "cell voltages" rather than "battery voltage".
So from here we only talk about individual cells and individual cell voltages. Since there are 4 cells in a 12V lithium battery it is tempting to multiply a cell voltage by 4 to get to the battery voltage, but unlike lead-acid batteries which are self-balancing, with Lithium batteries you cannot assume that all individual cells have the same cell voltage, especially not when the battery is almost fully charged or almost fully discharged. Hence the need to measure each cell voltage individually.
A few key values
A cell has only 10% left when the cell voltage drops down to 3.1V, and gets damaged if the Voltage ever gets below 2.5V. On the other side of the spectrum: the cell is 90% full when its voltage reaches 3.3V and gets damaged when it gets above 3.6V. You see, the cell spends most of its time around 3.2V so, except for a fully charged or fully discharged battery, it is impossible to tell its SOC by looking only at the Voltage. Oh, and to make it even more confusing: this nominal voltage varies with temperature!
How lithium cells work
A lot can be told about the exact chemistry of lithium batteries, but it requires more than average understanding of chemistry. Fortunately, it is not really necessary to know exactly how lithium batteries work; just a basic idea is sufficient to understand what happens when you approach or exceed any of the limits of lithium batteries.
Structure
Just like a lead-acid cell, a lithium cell has a positive plate (cathode), a negative plate (anode), some kind of mechanical separator between them and the whole is soaked into some electrolyte (conductive liquid). The anode of a LiFePO4 cell is made of a copper sheet coated with porous graphite. The cathode is made of aluminium coated with lithium iron phosphate material. The electrolyte consists of a lithium salt (typically lithium hexafluorophosphate, LiPF6) dissolved into a highly flammable organic solvent. Fortunately, the electrolyte is unable to leak out because it is fully absorbed in the plates and separator.
Charging and discharging
When a lithium cell is charging, the cathode which consists of LiFePO4 changes into FePO4 and the lithium migrates to the graphite anode to form LiC6. When discharging, the process is reversed.
Both the graphite and the iron phosphate are structurally very stable and don't suffer from the Lithium moving around, which is why these cells don't degrade from repeatedly charging and discharging.
Lifetime expectancy
Lithium cells can be virtually cycled indefinitely, lithium cells don't suffer from cycling! Lithium cells usually come to their due to one of the following causes:
- Exceeding limits. The manufacturers of lithium cells publish specification sheets with limits that should never be exceeded. There are voltage limits, charge and discharge rate limits, temperature limits, etc. In practice, limits are often exceeded and this causes degradation of the cells which will ultimately lead to their ends.
- Spending too much time in a highly State Of Charge. When the cell is somewhere between 80% and 100% charged, the chemistry within the cell becomes a lot more reactive, especially at elevated temperatures. This causes degradation of the cells.
- Lithium plating. This is caused by various factors, including excessive charge rates, cold temperature charging, or trickle charging (maintaining a high voltage for too long after charging should have otherwise been terminated). These cause lithium ions to be transformed into metallic lithium, typically on the anode, which results is in irreversible loss of capacity.
- Time. Whether you use the cells or not, the cells will loose capacity over the years due to internal degradation. The process is accelerated in higher temperatures; another reason to keep the cells as cool as possible.
Example specifications
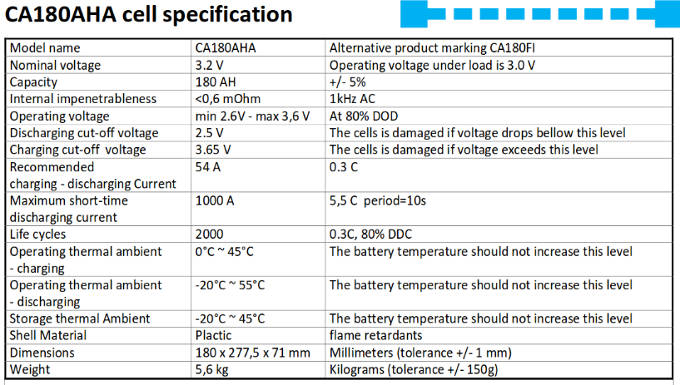
This is the factory specification sheet for the lithium cells used on ZwerfCat. We have eight of these cells: a set of two cells are wired in parallel and four of these sets are then wired in series. This results in a 12V battery bank of 360Ah capacity. Other brands have similar specification sheets, the numbers on them are quite similar, which is no surprise given the fact that all these cells use the same chemistry.
A few notes:
- The capacity is listed as plus or minus 5%. This means that there is a good possibility that there might be a difference up to 10% in capacity between individual cells in the battery. If you look at the Voltage curve we can see what a 10% in charge difference means for the cell voltages. The battery voltage might be still in the normal range while one of the cells may already be exceeding limits.
- Note the normal operating voltage and the "never exceed" values. For charging there is only a difference of 0.05V! For discharging there is a little bit more slack with 0.1V, which is still very small. If one would prefer wider margins this means less deep charging and less deep discharging, which results in less available capacity.
- The battery should not be charged below zero degree Celsius.
- Maximum sustained charge and discharge current is defined as 0.3C. This means 0.3 times the capacity. So for a cell of 180Ah this means 54 Amps.
Approaching and exceeding the limits
What happens when lithium batteries become empty?
The anode runs out of lithium and the cell voltage drops off very fast. To avoid damaging the cell, discharge should be terminated before the cell drops under 2.5V. Because of self-discharge and possibly discharge via the probe sensors or current supply of the Battery Management System, some reserve should be left, especially if it is anticipated that the cell will remain in a discharged state for quite some time (during the winter?). That is why it is recommended to store cells with about 40% of charge left.
What happens when lithium batteries are being overdischarged?
Allowing a Lithium cell to drop below 2.5V will not only damage the cell but it converts the cell into a timebomb. Due to unavoidable variations in capacity of the individual cells, not all cells are empty at the same moment. Keep in mind that in a series circuit, the current through the whole circuit is everywhere the same: If one cell is no longer able to produce the required current but the other cells are still feeding current through the circuit, the empty cell now sees a reverse polarity and copper from the anode starts dissolving into the electrolyte. When recharging this copper gets deposited onto the cathode where it forms very sharp dendrites which slowly grow and start piercing the insulation between anode and cathode. The subtle signs that this has happened is that the cell becomes warmer than normal when charging and that the cell displays a much higher self-discharge rate: Cells that discharge by themselves within a month or so are not healthy! A consequence is that such a damaged, self-discharging cell is now guaranteed to drop below 2.5V prematurely during the next discharge and the dendrites will consequentially grow some more. The cell is now on a pathway to catastrophic self-destruction: When the dendrites have grown large enough they will at some point completely short-circuit the cell and you just have to hope the cell will fail "nicely". Unfortunately, it appears that a cell which is damaged by overdischarging tends to self-destruct much more violently than a cell which is "normally" short circuited.
Overdischarging is the worst that can happen to a cell and turns it into a timebomb, so this must be prevented at all cost. A cell which has been allowed to overdischarge should be discarded, even if it appears to have "recovered". Consequently, cells showing a high self-discharge rate can no longer be trusted but should be discarded as well.
What happens when lithium batteries become fully charged?
Near the end of the charge, the cathode runs out of lithium and the cell voltage will increase very rapidly. The electrodes become a lot more chemically reactive and this slowly causes degradation of the cell. It is important to prevent lithium cells remaining too long in a fully charged state. It is even better not to fully charge them every time.
What happens when lithium batteries are being charged too fast?
Most manufacturers specify a maximum charge rate of 0.3C which means you are only allowed to charge with 0.3 times the Amphours (Ah) capacity of the cells. On our catamaran ZwerfCat we use two 180Ah cells in parallel, so we have a capacity of 360Ah. This means we should not charge with more than 108 Amperes. If a cell receives a charge current that exceeds the absorption rate of the cathode, the lithium ions are irreversibly transformed into metallic lithium which is then lost forever to the cells useful chemistry. This is called lithium plating. This problem becomes worse when charging at temperatures approaching 0 degree Celsius.
What happens when lithium batteries are being overcharged?
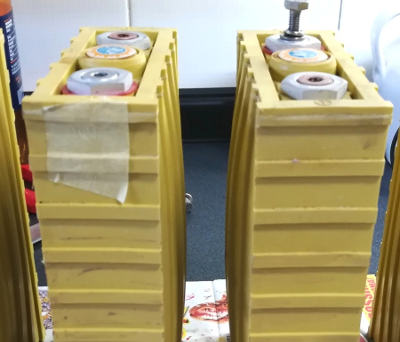
Lithium cells are not self-limiting; they keep accepting charge current even when they are fully charged. If the charge current is not terminated once the cell is fully charged, the cathode will completely run out of lithium and the cell voltage will keep increasing. At a cell voltage of 3.65V irreversible damage occurs. Quickly after that a cell voltage of 4.3V will be reached, from that point on the electrolyte starts decomposing into gasses. The safety vent starts venting and, if the current is high enough, the cell may even burst. If the charge current is substantial, the cell temperature rises to dangerous levels.
Note that due, to the voltage curve of lithium cells (which is flat over most of the trajectory but rises sharply when the cell is nearly full), it is entirely possible that when the first cell becomes fully charged its voltage suddenly rises above 3.65V, while the other cells are still at 3.4V. The overall battery voltage is then still at 13.85V which appears to be completely normal, despite the fact that one cell is already in destruction territory.
What happens when the temperature increases?
Lithium cells are chemically very stable, except when they are fully charged. The degradation which occurs when the cells are fully charged occurs much more rapidly when the temperature increases over 30 degrees Celcius. This is why it is important to prevent the cells from remaining too long in a highly State Of Charge, not to remain in elevated temperatures, and especially not both at the same time.
Thermal runaway
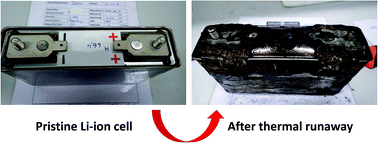
If the cell is heated above about 200 degrees Celsius, the oxygen bound within the iron phosphate gets released. This is extremely dangerous, because this free oxygen can then react with other elements in the cell, like the graphite and allow them to burn intensely. The resulting sudden extra heat releases more oxygen and thermal runaway is in progress. The fire most likely spreads to adjacent cells and, because of the additional internal oxygen released in the resulting fire, is very difficult to extinguish. Before spraying water over burning lithium cells: One of the vented components of the electrolyte may form hydrogen fluoride when it comes into contact with water, which is a very corrosive gas which can pass through the skin and is a known carcinogen.
Although the above sounds scary, this catastrophic scenario will only be reached when the cell is heated by some external means, like continuing to charge with a high current after the cell has become fully charged, or by a fire heating it up over the ignition temperature. Short-circuiting the cell or mechanically damaging it (like firing a bullet through it) will cause it to vent and/or burst, but not to ignite. Note: The latter is only true for LiFePO4 cells, other lithium-chemistries will be less forgiving.
What happens when the temperature decreases?
At temperatures around 0 degrees Celsius the lithium absorption rate of the cathode is significantly reduced and charging likely results in the formation of pure lithium metal, called lithium plating, and the lithium ions are then irreversibly lost from the electrolyte. Therefore, it is highly recommended to charge lithium batteries only at temperatures above 0 degrees Celsius. Discharging at lower temperatures is still safe, however the internal resistance of the cells tends to rise and the capability to support high loads may therefore be diminished.
What happens when not all cells in a battery-bank have the same State Of Charge?
When the individual cells in a battery don't have the same SOC we call that Imbalance. And imbalance is a problem because, when we charge or discharge a battery, each individual cell receives/gives the same current and is charged and discharged with the exact same amount of energy. Suppose a situation where one cell is 75% charged and another 25%. In this case, it is impossible to charge the cell with the 25% charge above 50% without overcharging the cell which was already 75% charged. The balance can only be restored by charging or discharging the cells individually. We call that Cell balancing.
What happens when you short-circuit a lithium battery?
Short-circuiting means connecting the positive wire of the battery directly to the negative wire, with no load in between. This might happen when the positive wire of the alternator becomes loose and dangles against the engine which is connected to the negative wire. There are many ways to create a short-circuit. If that happens...
You will have a very bad day. Seriously, a lithium battery has a very low internal resistance and it is thus able to deliver a tremendously high current! The cells can easily produce 10 times their Ah-capacity in short-circuit current, which on ZwerfCat means 3600 Amps! Most likely the wire which causes the short-circuit will evaporate, throw white hot molten metal and insulation around and possibly cause a fire. The cells will start venting violently, releasing toxic gasses.
Short-circuiting should simply not be possible: all wires need to be adequately fused. As an extra precaution, the positive wire from the lithium battery should incorporate a master-fuse, as short to the positive battery terminal as possible. On ZwerfCat we use a 400 Amp fuse, because we can't imagine a normal situation where we will draw at any moment more than 400 Amps of current. We might get close to this limit if we have the electric oven going, the anchor winch, flushing the toilet, etc. all at the same time, but I'd rather have to replace a fuse once in a few years than to extinghuish a fire.
Battery Management System (BMS)
As seen above, managing lithium batteries involves monitoring a lot of parameters and ensuring no limits are exceeded. Luckily, you don't have to do it yourself, a Battery Management System (BMS) can do this for you. A good BMS at least:
- monitors all individual cell voltages, temperatures, and battery current and provides some way of displaying and/or logging them
- facilitates charge termination when the battery becomes fully charged (only possible if the system is equipped with a power interrupter)
- facilitates discharge termination when the battery becomes fully discharged (only possible if the system is equipped with a power interrupter)
- alarms and disconnects the battery when it detects temperature anomalies.
- protects against low temperature charging
- reports or restores cell imbalance
Discharge termination
In the ideal world, the battery gets charged often enough so that it will never become empty. Just plug in the charger or start the generator/engine in time and you will be fine. But honestly, have you never experienced a flat battery in your life? Chargers might fail to charge properly, heavy consumers might fail to switch off, etc.
With a lithium battery, the battery should never ever be allowed to run flat or it will convert into a time-bomb!
As explained above, draining a cell completely empty will convert it into a time-bomb. If there is no BMS-controlled power interrupter preventing this from happening, the question is not if it will happen, but when it will happen.
With a Lithium system, you can't keep taking current from the battery until the lights get too dim to read a book. When a Lithium battery is depleted, the symptoms occur very abruptly. Due to the voltage curve of the Lithium cell, the voltage only starts sagging when the cell is almost completely discharged. It is almost guaranteed that at least one of the cells is fully discharged before the others, so the fatal voltage drop in the least charged cell/s would not be immediately obvious. Often there is not even an observer but a cell goes silently flat while the owner is asleep or away.
Due to the voltage curve of lithium cells and the fact that not all cells have exactly the same capacity, it is pretty much guaranteed that one of the cells suddenly drops in voltage while the other cells are still in the normal voltage range. Let's think about the situation where 3 of the cells are still at 3.1V and one is just about to enter the danger zone at 2.5V. The battery voltage would read 11.8 Volt which wouldn't be really alarming, and indeed, most equipment will operate just fine without complaining. Yet one of the cells is about to turn into a time-bomb.
The only solution is to incorporate a Battery Management System which monitors each cell voltage so a dangerous development will be detected in time, and the only thing a BMS should and can do is... disconnect the load from the battery with a power interrupter. Gone are the days of a graceful slow reduction in voltage, the lights going dim very slowly and navigation equipment starting to throw low voltage alerts to you, nothing notable will happen until the BMS very abruptly disconnects all the loads, leaving you suddenly in the dark and without navigation equipment. But that is better than to sail with a timebomb on board...
Charge termination
Lithium cells are, unlike lead-acid cells, not self-limiting when they become fully charged. If we keep supplying charge current, the cells will get destroyed. The charge must therefore somehow be terminated as soon as one or more cells becomes fully charged.
Voltage based charge regulation
It is shocking that there are manufacturers/vendors who choose this path. For lead-acid batteries it is a correct and common practice to define an end-voltage. This is encouraged by all the fancy chargers these days which allow different end-voltage settings for various types of lead-acid batteries. To make the list "complete" they often also offer a "lithium-voltage" setting. However:
It is impossible to regulate lithium charging by configuring a specific charge voltage!
Let's clarify this further.
If the cell voltage must not exceed 3.6 Volts and there are 4 cells in series, why not "regulate" the charge voltage to 4*3.6, which is 14.4 Volts? After all, most chargers have a setting for that.
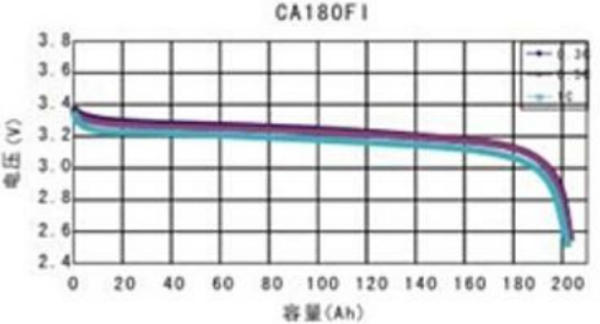
The problem is the charge curve of the Lithium cells. While charging, the voltage of a cell remains constant during the vast majority of the time and only starts rising abruptly when the cell is almost completely full. It is more than likely that not all cells reach this point at exactly the same time. If three of the cells are still at 3.5 Volt and one of it reaches 3.6 Volts (which is a difference in SOC of less than 1 percent!), then the point of damage is already reached when the voltage increases over 14.1 Volts ((3*3.5)+3.6). It is also entirely possible that one cell is still lingering at 3.4 Volts when the first one hits 3.6 Volts. So in reality the end voltage needs to be set even lower to remain on the safe side.
You might wonder why we do not do exactly that: setting the termination voltage at 4*3.4 Volts, which is 13.6 Volts? The same problem here: due to the voltage curve not all cells are guaranteed to reach 3.4 Volts at the same time: if we would use that as a cut-off point then some cells might still be at 3.2 Volt and that means that they might be less than half full when the charging is terminated. Even then it is still perfectly possible to destroy a cell: if 3 cells are still at 3.2 Volts and the "regulated voltage" is set at only 13.6 Volts then the remaining cell has to deal with 4.0 Volts, far into damage territory! So, "Voltage regulation" should never ever be used when charging Lithium batteries. (Yup, I know, your wonderful charger has a "Lithium setting" but that is just the result of ignorance or marketing by the manufacturer; battery voltage regulation simply cannot work with lithium batteries.)
Then what about keeping the cells perfectly balanced, so that they all reach the same voltage at exactly the same moment? Again, this is not so simple and reliable as will be explained in chapter Cell balancing. Also, due to the very sharp voltage rise at the end of the curve, very small differences in cell capacity/balance result in a substantial voltage difference at the end of the charge curve. For now, let's conclude that, unlike with lead-acid batteries, (repeat with me:)
it is not reliably possible to regulate lithium charging by configuring a specific charge voltage.
So, the only remaining options are to either interrupt the current path from charger to battery or somehow instruct the charger to stop outputting current.
Interrupting the current path from charger to battery
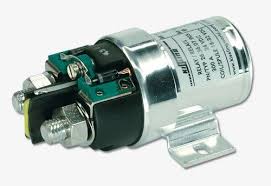
That would be simple: just unplug the battery once one of the cells hits the termination voltage. Of course you don't have to physically unplug the battery, but the task can be carried out by a BMS commanded relay. On a ship however, there are a few complications with this approach. A standard alternator will surge if you disconnect the battery, fry itself and take the delicate equipment of the boat with it in the process. Disconnecting a Lithium battery from the alternator can only be done safely if a lead-acid battery is left in place. The same applies to wind generators and solar installations: they are not designed to operate without a battery permanently connected to them.
There is also the question what to do with the equipment of the ship when the batteries are fully charged and the path between the charger and battery is interrupted:
Keep the equipment attached to the charger
In case the equipment stays attached to the charger, there is no longer a battery to act as a buffer (it got disconnected because it was fully charged) and if a load that exceeds the maximum current of the charger is switched on this will immediately result in a severe voltage drop which will likely result in equipment reboots or malfunctions. It is generally a bad idea to feed equipment directly from an alternator or other charge source, without a battery somewhere in the circuit to provide a buffer.
Keep the equipment attached to the battery
If you choose to leave the equipment connected to the battery, this means that, with no charge source connected to it anymore, the equipment starts drawing from the battery right away, even if there would still be plenty of charge current available. So, in practice, once the solar charger has fully charged the battery, it gets disconnected from the battery, and the on-board equipment immediately starts drawing from the battery and the solar charger is no longer doing anything.
Instructing the charger to stop charging
This is a more sensible approach. This is what Tesla and most other lithium chargers do: just shut down the charger. Easy peasy. On a ship however, it involves replacing the alternator (or its controller) with a special one which communicates with the BMS and interrupts the field current to stop charging while it keeps running. Something similar should be done with the grid charger, the solar charger, wind generator and diesel generator. You are likely going to have to replace all these chargers, run communication wires to the BMS, remember later when you add or replace a charger, or use a portable generator(!) to have it communicating with the BMS as well, and greatly complicating the system and adding multiple potential failure points in the process. Even then it is still highly recommended to have a power interrupter between the battery and the rest of the system, to be able to interrupt the charge current the hard way if one of these chargers no longer obeys the command to stop charging. The resulting power surge will fry the equipment on board but that is still better than a bursting lithium battery and still frying all the equipment.
Also, the problem still remains that, with the charger correctly powered down, the equipment of the ship immediately starts drawing from the battery, even when the (now shut down) solar charger would have been able to feed the equipment.
Interestingly, it could in theory be possible to "modulate" the charge current in such a way that the current to and from the lithium battery remains zero. This way it could be possible to create a "float" condition where the charge sources just provide enough power to feed the equipment but no current goes in or out of the lithium battery. However, I'm not aware of any BMS (with dedicated charge sources) which has implemented this, and it would involve even more complicated modifications on all the charge sources.
Hybrid systems
All the above sounds very unsatisfying and frustrating? Well, there is a simple and reliable solution: Just keep the good old lead-acid battery in place and have the Battery Management System disconnect the Lithium battery once one or more cells are fully charged. The still connected lead-acid battery acts as a buffer both for chargers and for power surges. The alternator and other chargers are happy because they still "see" a lead-acid battery connected to them. Nothing has changed from the original setup; the wiring and chargers can continue to work as designed. The fully charged lithium battery is simply "parked" aside when it is full, waiting to be brought online again when the power demand of the ship exceeds the available charge current; in the mean time, the equipment of the ship is powered from the chargers as usual with a lead-acid battery to smooth things out, just like in the old days. You only need a BMS which is designed to work with such a hybrid battery system. Read more about the advantages of lead-acid/lithium hybrid systems.
Dual bus topology
If a lithium-only installation is used, to enable the BMS to disconnect the chargers and load separately (which is the only sensible way to operate such an installation), it is necessary to split the main DC-bus into a "chargers branch" and a "loads branch". This may, depending on the previous implementation of the wiring, be a substantial and expensive rewiring task, especially because heavy gauge wiring with crimped terminals must be used. A few notes on this:

- Some devices may not entirely fit into either a "charger" or "load" category. For example, a solar charger usually provides current output, but during the night, it might actually consume some electrical power to keep its internal electronics running. If the BMS disconnects the loads because one of the cells has become fully discharged, the solar charger is on the "charge branch" and does not get disconnected, while it continues to draw power, dragging the fully discharged cell into destruction.
- Splitting the bus into a "charge branch" and a "load branch" is not very intuitive, especially not if devices are spread over the ship, and likely to invite errors in the future. If someone else works on the installation in the future, to, let's say, install a water maker, that person might find a very comfortable 12V wire to connect to (yup, the color is red and indeed it carries 12V), while it may be the 12V wire routed to the charge branch. The newly installed watermaker might then be able to draw the battery completely flat and change it into a timebomb while the BMS is unable to do anything about it simply because it is on the wrong branch...
Cell balancing
When the individual cells in a battery don't have the same State Of Charge we call that Imbalance. And this is a problem, because when we charge or discharge a battery, all cell which are connected in series receive the same current and are charged and discharged with the exact same amount of energy. The balance can only be restored by charging or discharging some of the cells individually.
How can cells become imbalanced?
Actually, in a healthy battery with cells from the same production batch it is not very likely that the cells become imbalanced anywhere soon. Imbalance is not the result of charging or discharging, because by physical laws the current through all the cells in series will remain exactly the same. Imbalance occurs when one cell has a higher self discharge rate than its siblings, which should be nearly negligable. If the difference in self discharge between the individual cells is substantial enough to require frequent balancing, something is seriously wrong with one or more cells.
Restoring the balance
When lead-acid cells become imbalanced the solution is to just keep charging until all cells have reached 100%. The cells which reach the 100% mark first simply use the excess energy to convert some water into its components hydrogen and oxygen, and the lost water can be replenished (wet cells) or reclaimed (maintenance free cells).
For Lithium cells this is more complex, as they can not be overcharged without getting damaged and the charge attempt has to be terminated when the first cell reaches 100%. Balancing cells can only be done by putting a load over individual cells, discharging only a particular cell, until that cell has the same State Of Charge as its neighbours. A sophisticated BMS can perform this task automatically but don't expect too much of it. It is hard if not impossible to do right with a battery which is still connected to a charger or a load, due to the varying internal resistance of the cells: A cell might appear to have a higher voltage during charging because measuring takes place outside of the cells. Also, according to the example specifications the nominal voltage is 3.2V but sags to 3.0V under load. If the battery is not disconnected then a voltage inspired balance attempt is likely to actually destroy the balance of the cells. Therefore, a BMS should only attempt to balance the cells after careful observation of multiple charge/discharge cycles, after receiving permission from the user, when the battery is disconnected, and only as a maintenance routine no more than a few times per year. If a battery is not able to maintain its balance for a long time, this indicates a higher self discharge rate in one or more cells, possibly due to internal micro shortcuts caused by overdischarging, and for your safety you should consider replacing the battery instead of rebalancing it frequently.
Top or bottom balancing
It is unlikely that cells in a battery have exactly the same capacity (see the example specifications). So it is possible to balance the cells so that they reach the fully charged state at the same moment, or that they become empty at the same moment. The former is called top balancing, the latter is called bottom balancing. It is impossible for the cells to both become fully charged at the same time and also become fully discharged at the same time, if the capacities are not exactly the same. Since on a sail boat the cells are more often routinely fully charged than fully discharged, it makes more sense to top-balance the cells. The margins are narrower at the top end than on the bottom end and this further promotes top-balancing. As far as I know all BMS's for sailboats on the market use top balancing. So BMS attempts to balance the cells in such a way that they reach a full SOC at roughly the same moment, and during discharge it will be the "smallest" cell that will pass out first and trigger the discharge termination.
Active versus Passive balancing
"Active" sounds better than "passive" but it is not worth it for our purpose. Passive balancing simply means converting the surplus capacity from the fullest cell into heat, whereas active balancing means using the surplus energy from the fullest cell to help charging the other cells. The only benefit of the latter is that less energy is wasted, but since the imbalance should be very small and not occur very often, the advantage is questionable. The disadvantage is that the electronics to do this are quite complicated, prone to failures and expensive. There is simply no good reason for this in a boat where the charge currents tend to be relatively high and one amp extra charge current really doesn't matter. For its intended purpose; restoring the cell balance, passive balancing is just as good as active balancing.
Terminology
Relay, disconnector, interrupter
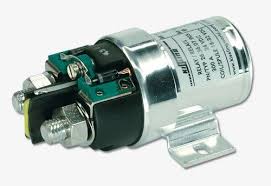
These are all names for a device which can interrupt the current, controlled by an electrical signal. These are similar to manual master switches, except that the switch is commanded by an electrical signal. This way, a small control signal from the BMS can switch off the all the loads or chargers at once, regardless the amount of current.
SOC (State Of Charge)
State Of Charge is expressed in percentage, where 0% means completely discharged and 100% means fully charged. Note that SOC is the opposite of DOD: After a DOD of 80%, an SOC of 20% remains.
DOD (Depth Of Discharge)
Depth Of Discharge is expressed in percentage. With lithium batteries, a DOD of 80% is common. For lead-acid batteries, a DOD of 30% is more appropriate. Note that DOD is the opposite of SOC: After a DOD of 80%, an SOC of 20% remains.
CCL (Charge Current Limitation)
Charge Current Limitation is a function of the charge source which limits the maximum available charge current. OpenHybridBMS implements a softfuse which can disconnect the lithium battery if the charge current exceeds a configurable parameter.
DCL (Discharge Current Limitation)
Discharge Current Limitation can be implemented by a simple fuse or as a function of the BMS. OpenHybridBMS implements a softfuse which can disconnect the lithium battery if the discharge current exceeds a configurable parameter.